Atomically-controlled Quantm Oxides
Atomic-scale or nano-scale heterostructure
engineering in IV, III-V and II-VI semiconductors, and elemental
metals has led to many discoveries and developments such as fractional
quantum Hall effect, semiconductor lasers, giant magnetoresistance
and magnetic tunnel junctions. The key player behind
this success is the Molecular Beam Epitaxy (MBE) technique, with
which one can grow arbitrary heterostructures of atomic-precision.
One of the main efforts in our group is to extend the realm of this
proven technology to a newly-emerging and less-explored material
system, the complex oxides.
Complex
oxides exhibit much more copious electronic properties than do the
conventional semiconductors and the elemental metals, such as semiconducting,
high Tc superconducting, colossal magnetoresistive, (half-)metallic,
(anti-)ferromagnetic, ferro(para, piezo, pyro)electric, and multiferroic
behaviors. Furthermore, researchers have already demonstrated that
when two complex-oxide systems are interfaced with each other, multifunctional,
or sometimes completely new, properties tend to emerge. This suggests
that if we apply the atomic-scale heterostructure engineering scheme
to this complex oxide material system, we may discover many more
novel properties and applications.
However,
compared
with the conventional semiconductor and metal MBE's, complex-oxide
MBE requires much higher level of technical sophistication. In order to tackle this problem effectively,
we have built a unique oxide-MBE system , and utilizing the new oxide-MBE
system and drawing on the strong theoretical and characterization
support of other Rutgers groups, we will synthesize and study nanostructured
oxide "metamaterials" and search for novel functionalities
in these emerging territories.
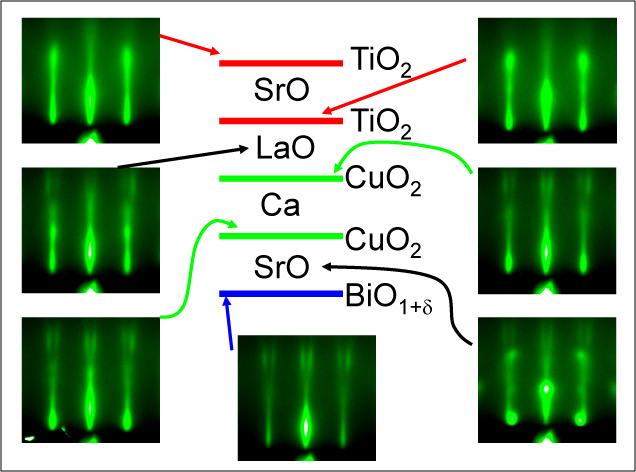
Figure: Reflection High Energy Electron Diffraction (RHEED)
images
showing atomic-layer sequence of an artificial complex-oxide
interface
Nano/micro Oxide Devices
As
complementary tools to the atomic-precision oxide-MBE technique, we
make use of other thin film growth techniques such as PLD (Pulsed
Laser Deposition), CVD (Chemical Vapor Deposition),
Ion Beam Assisted Deposition (IBAD) and Sputtering. Each of these
techniques has its own advantages for certain applications, and we
utilize each technique for different applications.
On
many of the thin film systems created by one of these growth techniques,
we routinely create nano/micro-device structures by relevant lithography
techniques and investigate their electronic properties such as strongly-correlated
artificial 2D electron gas, artificial high Tc
superconductors, magnetism-superconductivity
proximity effect, artificial ferroelectrics and multiferroics, ferroelectric/magnetic
tunnel junctions, electric/spin field effect, and spin- injection Josephson effect.
Materials Research
for Superconducting Quantum Computers
In
parallel with the oxide metamaterials efforts, we also conduct materials
research for superconducting quantum computers. Superconducting quantum
computers based on Josephson junctions have been the recent focus
of many researchers in quantum computation due to their scalable architecture.
However,
as Prof. Oh and his collaborators have demonstrated, amorphous
materials routinely used for the tunnel barriers and the wiring
dielectrics are a major source of decoherence, which seriously deteriorates
the performance of superconducting quantum computers. This suggests
that it is essential to develop highly coherent materials before
realization of practical superconducting quantum computers.
As
a pioneer in this field, we are investigating the role of material
disorder as a decoherence mechanism and searching for advanced materials
for coherent superconducting quantum computers. This project is
a joint effort with National Institute of Standards and Technology
(NIST), Colorado.
|